Ships that sail the seas foul to slime. What do we know about this universal fouling problem?
Firstly, we know that the marine industry is not alone in facing a slime challenge. As fouling control coatings researchers at AkzoNobel, we are interested in the drag consequences of biofilms (slime) on ships. Surgeons worry that biofilms on implanted medical devices can harbour pathogens, or that biofilms in chronic wounds result in long lasting infections. Biofilms in dairy plants can result in food spoilage. Accumulated biofilms in power plant cooling towers can drastically reduce heat exchange. Biofilms can reduce the cross-sectional area of pipes, resulting in drinking water transport inefficiencies or blockage of fuel lines. Dental biofilms lead to painful tooth decay and other health problems. And biofilms are even a potential problem in space, where they might act as hotbeds of microbially induced corrosion on long term installations like the International Space Station.
Whether marine, clinical, industrial or extra-terrestrial, biofilms are characterised by properties that give them superpowers of persistence which are especially vexing in industrial contexts. A biofilm is made of living microorganisms embedded in an extracellular polymeric matrix produced by some or all of the cells, and typically is adhered to an immersed surface. The microbial community diversity can range from biofilms dominated by only a few species of bacteria to highly diverse environmental biofilms that contain thousands of species of bacteria, microalgae, fungi, protozoa and other species all living in close association. The architecture, chemical composition and physical properties of biofilm matrices are likewise variable, reflecting the species that form the biofilm and the local environmental conditions such as local flow conditions. Finally, these diverse microbial systems can also respond to environmental change on timescales of minutes, hours, and days, modifying their biological, chemical, and physical properties.
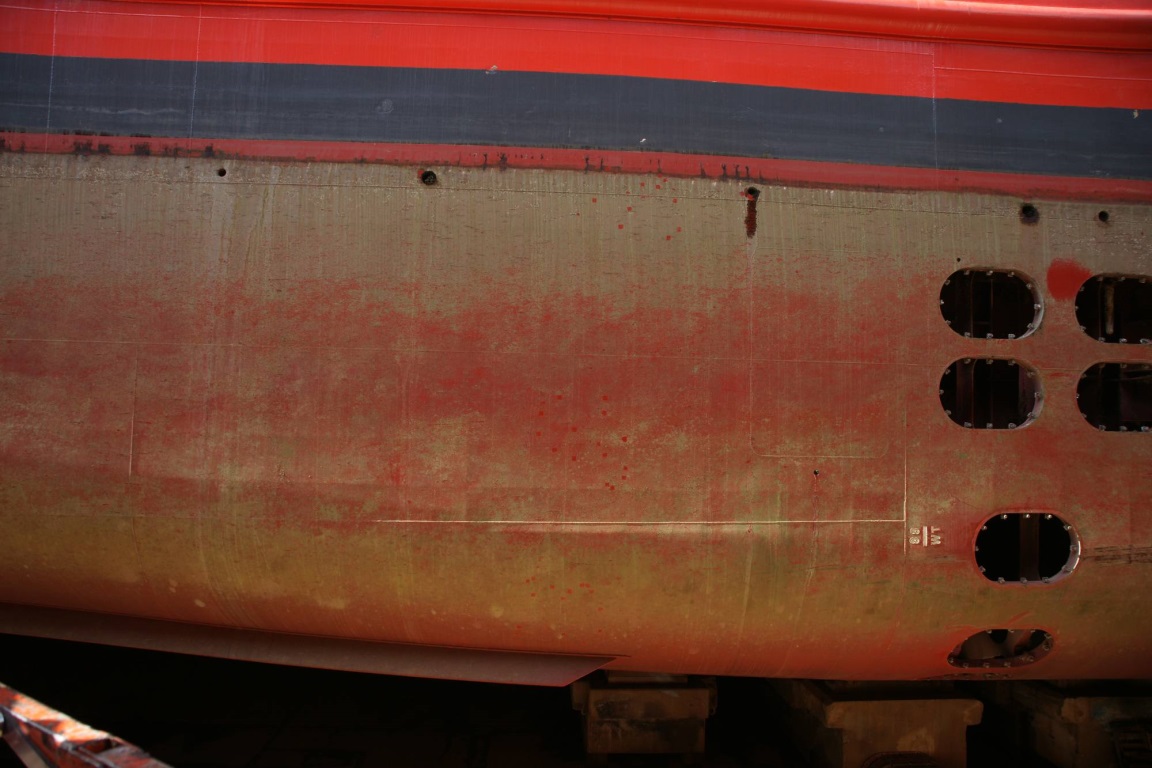
FIGURE 1
Ship slime is a smart microbial lifestyle: microalgae, bacteria and other microbes produce an extracellular matrix that adheres the microorganisms to the surface, and provides cohesion within the biofilm and protection from mechanical and chemical disruption.
The matrix also shields its microbes from chemical attacks by antibiotics or biocides. In some instances substances in the matrix will directly sequester chemical agents, while in other cases the matrix provides a simple physical diffusion barrier, where less and less of the chemical actually moves into the deepest areas of the matrix. Cells inside this deepest, most protected zone of the matrix may be effectively starving and fall dormant, because nutrients would also have to cross the diffusion barrier. However these dormant cells are a kind of microbial reservoir, and should all the other cells die and the outer layers of the biofilm disintegrate, the inner cells may jumpstart the community all over again and refresh the biofilm. The role of the matrix as a chemical shield has been proposed as a contributing factor as to why slime grows on biocidal marine coatings.
Given the remarkable resilence of biofilms to physical and chemical stresses, combines with the global diversity of marine microbes, biofilms can reasonably be expected to grow on any immersed marine coating over time in real world vessel operating conditions. However, if you survey the hulls of newly dry-docked ships around the world, ot the experimental coating panels that have been immersed at our field testing sitesm you would intuitively observe that not all fouling biofilms are the same, They vary in colour, texture, spatial coverage and thickness, and these visual cues indicate there might be further differences in slime microbial communities and in the frictional drag penalities attached to different biofilms. The AkzoNobel fouling control marine biologym hydrodynamics and coatings research teams are incorporating investigatory methods from across the multi-disciplinary fields of biofilms science to better understand the varied properties of biofilms that form on marine surfaces and, in parallel, improve our coating development processes. Within this context, we are asking whether there is a biological slime metric that is a robust predictor of the associated slime drag penalty.
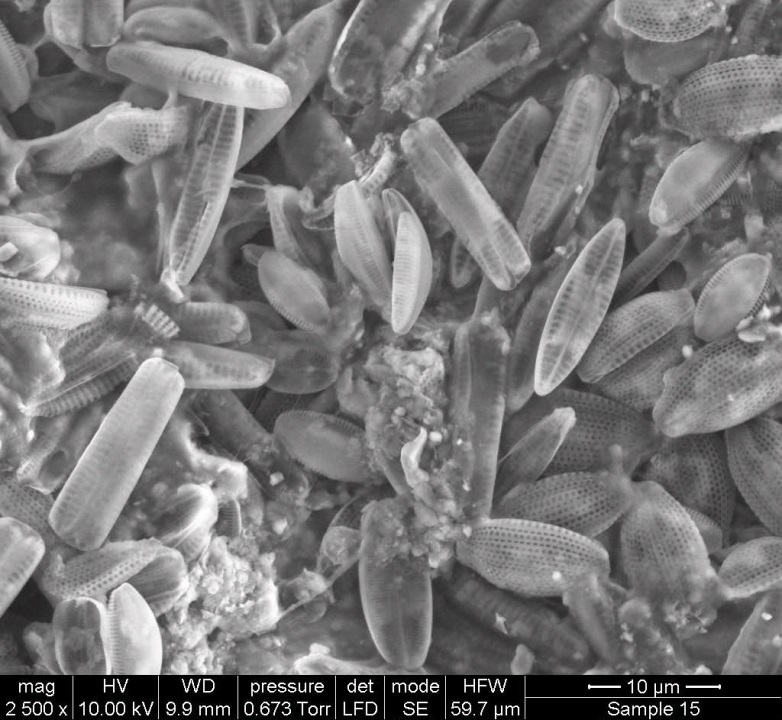
FIGURE 2
Environmental scanning electron microscopy reveals the often beautiful morphological detail of diatoms, single celled microalgae that are typically major constituents of marine fouling biofilms.
For the biologists in the team, a natural first approach is to examine the communities in fouling biofilms. Marine ship biofilms are generally comprised of both bacterial and microalgal populations, and one of the most recognizable constituent microbial groups is the diatoms. These single microalgal cells are encased in intricate silica shells and can be identified by shell morphology. We’ve used both environmental scanning electron microscopy and standard light microscopy to analyse biofilm samples collected from ships and have catalogued the slime diatom diversity at different hull locations, definitively demonstrating that ship slime varies along and down the hull and can vary from ship to ship and coating to coating.
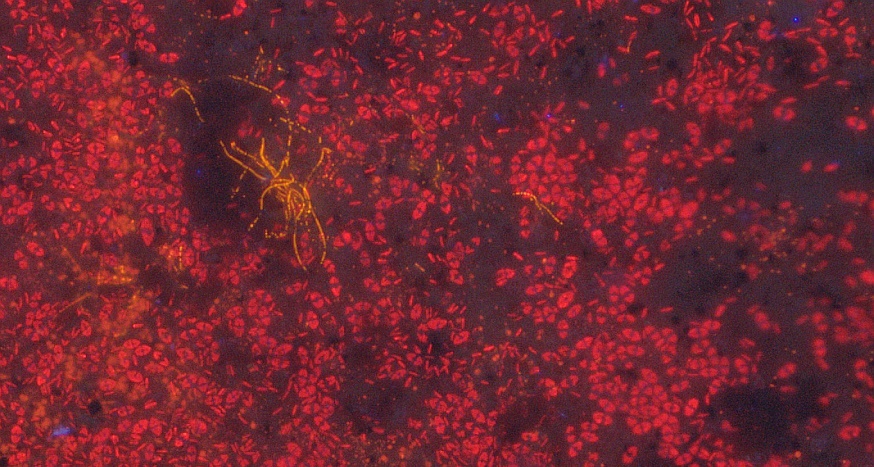
FIGURE 3
Microalgae in marine photosynthetic biofilms auto-fluoresce in signature colours, reflecting their individual photosynthetic and accessory pigments
Though diatoms are common in fouling marine biofilms they are by no means the only microalgae present. All microalgae contain pigments which they use to convert light to energy by photosynthesis and additional pigments which play other associated roles and are often specific to a particular type of algae. Measurements of microalgal pigments in plankton samples are regularly used in oceanography as broad fingerprints to identify the different algal groups present, and we’ve adopted the same approach to characterise slime. Biofilm pigment chromatography analysis has revealed that in at least some experiments, several coatings are well-colonized by a particular type of microalgae that is effectively absent from other coatings. Another property of microalgal pigments is that they auto-fluoresce – that is, the pigments capture shorter wavelength light, absorb some of the energy, and then emit light at longer wavelengths. Different pigments fluoresce at different wavelengths, so we can use epifluorescence microscopy to confirm visually the output of pigment chromatography analysis. For example, under an epifluorescence microscope with a UV/blue light source, diatoms and cyanobacteria, which will auto-fluoresce deep red and orange respectively, are clearly distinct.
Metagenomic analysis is a more finely resolved technique for obtaining a broad overview of the pooled microbial community in a fouling biofilm. We’ve been partnering with university microbiologists and bioinformaticians to study how bacterial diversity and total fouling community diversity reflect the influences of the underlying coating as well as biofilm maturity. AkzoNobel biology team researchers have extracted DNA from hundreds of slime samples, and each new result offers an exciting insight as to how communities form.
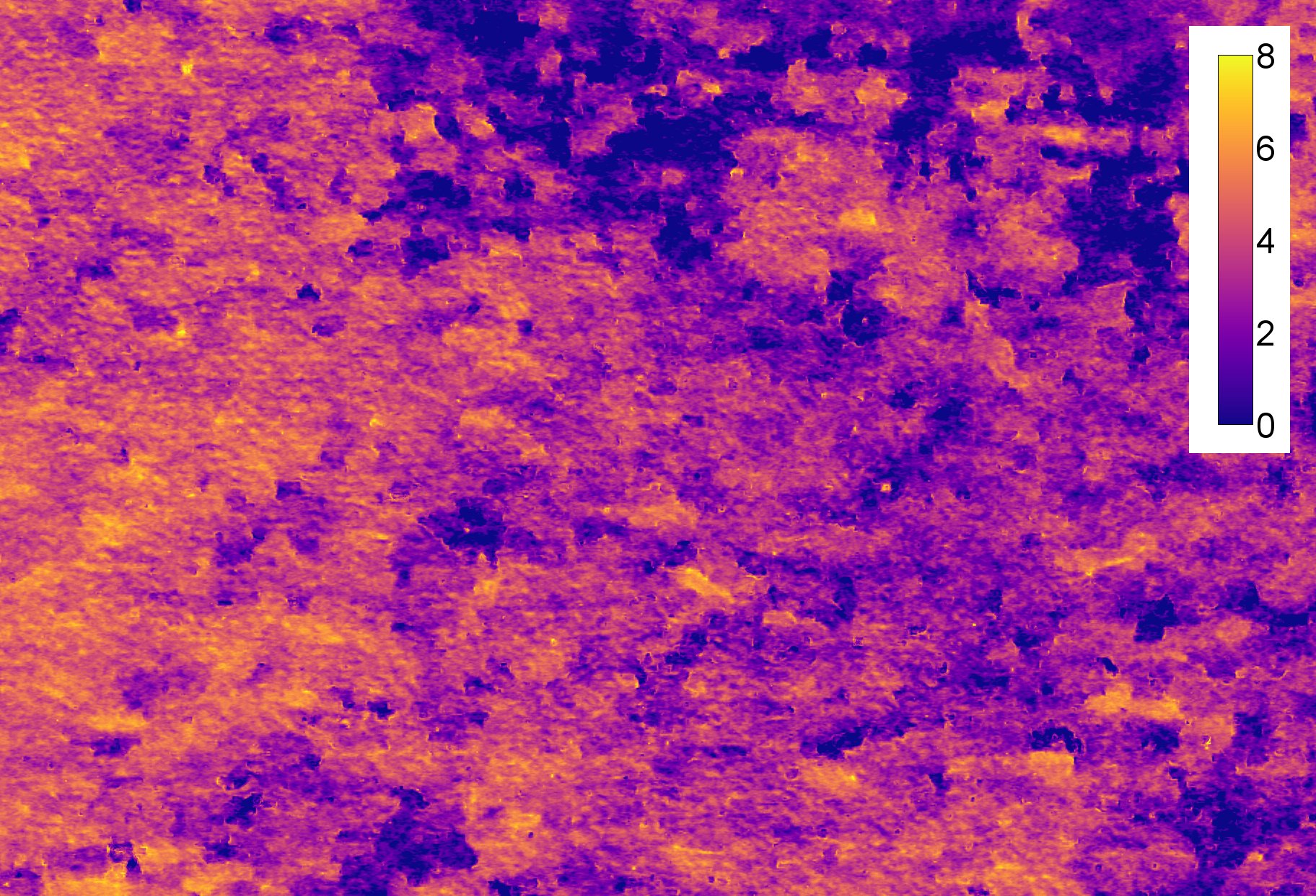
FIGURE 4
Multispectral imaging approaches to mapping biofilm density, such as this map of chlorophyll surface area density of a slimed panel, have roots in satellite sensing of global vegetation and ocean productivity.
We also want to be able to link biofilm properties with reliable and objective measurements of how much biofilm is fouling a surface. To estimate total biomass, we’ve once again taken advantage of the fact that most ship slime contains photosynthetic, pigmented microalgae. In oceanography, the amount of chlorophyll in a body of water is a long-established proxy for the amount of photosynthetic microalgal biomass. Chlorophyll absorbs red light and reflects green light, and likewise has characteristic absorption properties for other light wavelengths. If you can measure how much light is absorbed and reflected by photosynthetic biomass, you can approximate how much biomass there is. Multispectral biomass imaging, as this approach is called, is how the global marine microalgal conversion of sunshine to biomass is mapped from space by satellites. We’ve been developing similar methods to benchmark coating performance in lab assays, immersion trials, and on ships, and are looking at not only how much biomass is in slime, but how the biomass is distributed across the surface of a ship.
How do biofilm biological characteristics link up with biofilm physical properties like thickness, surface roughness, texture, and viscoelasticity? It is a challenge to measure the surfaces of biofilms so as to characterise their physical properties over appropriately large scales. The go-to methods for coating or biofilm surface analysis all have limitations when it comes to biofilm: laser light can be reflected and scattered from a biofilm surface, indentation methods can deform a biofilm, and microscopy can only image very small surface areas. In very recent years, though, biofilm structural research has opened up with the adoption of an imaging technology called optical coherence tomography (OCT). OCT lets us image the surface and interior structures of living biofilms over a surface area of approximately one square centimeter. For the last three years we’ve been using OCT imaging to get a closer look at the biofilms that grow on different coatings, and have developed methods to convert image data to biofilm topography, thickness, biovolume and surface roughness data. OCT video imaging is also possible, and we’re exploring how fouling biofilms on different coatings respond structurally to different water flow rates.
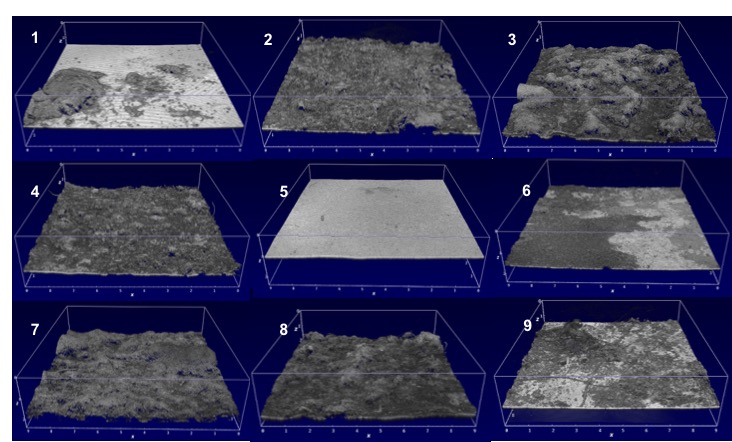
FIGURE 5
OCT imaging reveals highly visible differences in slime topography for biofilms grown over 13 months on 9 different coatings, including both biocidal and non-biocidal coatings.
Again, one of our main objectives is to develop biological and physical predictors of biofilm drag so as to incorporate those metrics into coating development. In parallel with exploring biological techniques, the hydrodynamics and marine biology research teams have been developing both in-house and collaborative approaches to measure the drag of slime on coatings. With flow cells and rotating disc systems, collaborative projects and in-house experiments, we are working to understand which methods are best suited to tie slime hydrodynamics and biophysical properties together.
Biofilms are natural super-structures and are not an easy challenge to address, which is why we are actively applying the investigative methods outlined here and other techniques from across the diverse field of biofilm science in our development of slime-resistant coating technologies.
@JenniferLongyear (Technology Leader l, AkzoNobel Marine Coatings)
Contact us
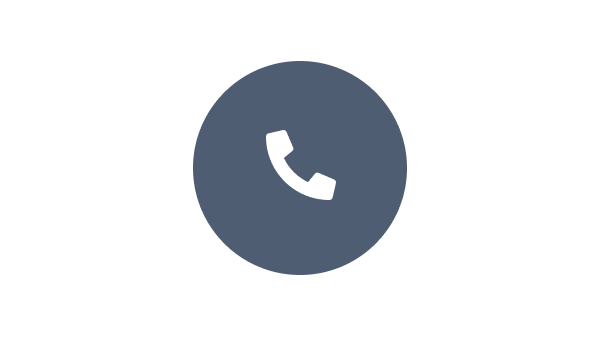
We are here to help
Speak with one of our experts to discuss how we can get the best results for your area of application.
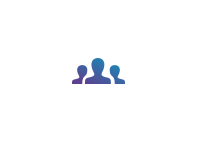
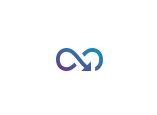
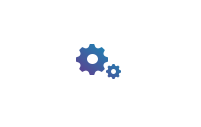